Typical Expected Change Rates in qEEG Resulting From Neurofeedback Training
Richard Soutar, Ph.D. BCN, John Hummer, Ph.D. BCN
New Mind Technologies
Atlanta , GA, USA
Abstract
Very little is presently known about the expected amount of change over time as a result of neurofeedback training, as well as the potential impact of clients’ physiological health status upon expected rates of change. To explore these issues, the pre-intervention, mid-intervention, and/or post-intervention qEEG data of 477 clients who completed standard or extended courses of neurofeedback was drawn from the New Mind Database, with neuro-metric indices contained in the pre-post qEEG maps analyzed for percentages of movement toward the reference norm (aka normalization) and percentages of movement away from the reference norm (aka re-organization). Statistical analysis revealed the global average percent of change (total change) across all neuro-metric dimensions for 20 neurofeedback sessions to be 38%, with change rates toward the norm complimentary to change rates away-from-the-norm. Rates of change were similarly analyzed for 20-30 sessions, 30-40 sessions, and 40-60 sessions of neurofeedback. Physiological health conditions had only a modest impact upon the various rates of change.
Introduction:
For well over two decades, qEEG (quantitative encephalography) has been the assessment standard in the field of neuro-therapy (neurofeedback). Surprisingly, many important questions remain unanswered regarding fundamental aspects of change, including the expected rate of change over time, on average, among clients undergoing standard (20 – 40 sessions) and extended (40 – 60 sessions) courses of neurofeedback (Soutar, 2014). Methodological and substantive issues needing to be addressed include the following empirical questions:
-How much change can we typically expect in a pre-post qEEG when doing neurofeedback and how does that compare to individuals not doing neurofeedback?
-What would be considered ‘abnormally high’ and ‘abnormally low’ amounts of change to be expected?
-Do individual health factors affect the rate of change during neurofeedback?
-How much do differences in time of day when the qEEG is recorded bias the amount of difference between pre and post qEEG brainmaps?
-What metrics should we use to assess change and how should they be calculated?
-At what point should we remap and how often should we remap?
-Is there ‘good’ change and ‘bad’ change and how do we tell the difference?
Historically, sources of research funding to address such important questions have been scarce to non-existent. Current conventional judgements regarding these questions derive from clinical opinions based upon experience and observation. Yet, subjectivity and other confounds limit the accuracy of any inferences or predictions that can be drawn. Potentially biasing factors that have not been controlled for include the clinical status of clients (‘treatment seeking’ client selection issue), variable symptom constellations, variability in client diagnosis, age, gender, medication status, dietary and sleep patterns, among other variations across clients. In addition, the type of protocols used, determination of training location(s), consistency of training and a host of other training-related confounds enter the picture. As expected, a wide variety of opinions arise from experience due to the impact of these confounds and the wide range of individual responses. Of further concern is the impact of artifacts on the analysis and the related quality of the map record.
An additional confound that is easily overlooked is the lack of standardization among map systems (the small number of proprietary large client databases). Comparisons between two different brain mapping systems are very difficult to make unless clinicians are highly trained in signal processing, neuro-metrics and statistics (Hammond et al, 2004). Most practitioners lack the background to make determinations based on this specialized knowledge base. As mentioned above, lack of standardization in protocol determination also confounds research and analysis in this domain.
In an effort to begin to explore possible answers to these questions, New Mind developed a database system that standardizes map interpretation and protocol determination. In addition it collects information on client health, age, and gender as well as many other potential confounds. The New Mind Training software can be cross-referenced to the qEEG maps through an anonymous client ID system that cannot be referenced back to actual client identity according to NIH standards. All data is anonymized and scrubbed in accordance with these standards.
The resulting data (derived from a large clinical data pool) allows us to draw samples of standardized pre-post maps that were generated using standardized protocols from over 1000 clinics. Although we are not drawing subjects from a random national sample, which is unrealistic under the circumstances, the number of clinics and diversity of national locations as well as the number of subjects begins to offset some of the confounds involved. It also begins to allow us to assess the impact of several of the more salient confounds, especially physiological health status.
Clinicians have traditionally based their judgements of positive outcome upon client self-report, or symptom assessment measures. Less common are efforts to operationalize change empirically, such as via qEEG re-assessments conducted at intervals during a course of intervention (mid qEEGs), and finally again at the conclusion of intervention (post qEEG). While most clinicians utilize an initial brainmap (pre qEEG) as part of their initial evaluation and protocol selection, it is far less common for clinicians to conduct intervallic brain maps (mid-qEEG) to ascertain progress and protocol effectiveness/adjustments, as well as to acquire an end-of-intervention brain map (post-qEEG). The time and expense involved in performing successive qEEGs is often prohibitive, yet such repeat assessment would appear requisite to operationalizing functional changes in the brain as a result of protocol-based training.
Even in instances where pre/post qEEG data measurements have been collected, there are no operational guidelines or specifications regarding which (or which combination of) neuro-metric component variables should be used as reliable and valid change measures.
Effecting functional neuroplastic change via neurofeedback is a non-linear process(Freeman, 2005; 2009; Soutar, 2014), just as psychotherapeutic processes appear to be (Hayes, et. al, 2007). In addition to symptom improvement, increasing the brain’s adaptive neuroplasticity is a primary goal, as is limiting the level of inevitable non-adaptive neuroplasticity where possible. Neurofeedback-induced neuroplastic change is less likely to resemble a positive slope on a graph, and more akin to solving a Rubik’s cube. One does not immediately come to a solution, but must shuffle components in a successive (and hopefully strategic) sequence in order to solve the puzzle. Client’s seeking neurofeedback present on initial qEEG with neuro-metric indices that are statistically deviant (in the positive and negative directions) from the population norm(s). Solving the puzzle in neurofeedback terms strives for (but does not always result in) movement of these indices in the direction of, and hence closer to, the statistical population norm(s).
The strategic process involving incremental improvement (toward the norm) could be termed adaptive neuroplastic change or ‘normalization’. Moving around components (resources) away-from-the-norm, but with which to hopefully facilitate change over time toward the norm could be termed compensatory processes of functional ‘reorganization’, as defined by Pascual-Leone (2005). ‘Global’ change (a composite measure of change due to normalization and change due to reorganization, summarizes the entire change process as assessed neuro-metrically. The New Mind qEEG Brainmapping System has empirically derived algorithms (including neuro-metric indices most sensitive to change) to enumerate percentages of normalizing organization and compensatory re-organization, summarized as Global or Total Change when comparing sets of qEEG data (e.g. pre/mid, mid/post, pre/post qEEGs).
As aforementioned, the neurofeedback Scientist/Practitioner is faced with the reality of in-availability of research grants and other funding sources from which to undertake stratified random sampling of treatment-seeking and non-treatment-seeking clients in the general population and performing serial qEEG re-assessments (following pre-qEEG). A reasonable and enlightening alternative is to draw client data from a large qEEG database derived from numerous clients/providers/clinics. One important parameter – duration of intervention –can be determined for both standard (20 sessions; 20 – 40 sessions) and extended (40 – 60 sessions) lengths of intervention. Additional parameters that can be derived include change measures (% normalization, % re-organization or compensation, and overall or total % change), as well as a composite index of self-perceived health problems, to be described in the Methods section.
By sampling broadly across a wide variety of clients and conditions, change variability that can ostensibly be attributed to the active intervention (neurofeedback) can be analyzed. Findings and conclusions are limited to intervention-completing clients, and not to the general population (of both treatment-seeking and non-treatment-seeking individuals). In summary, despite a potential selection bias (inclusion of those individuals who can afford and seek neurofeedback intervention, and complete it without prematurely dropping out), the New Mind Database System (Soutar, 2014) would appear to be acceptable and very informative archival source for conducting exploratory analyses to begin to estimate some of the aforementioned parameters. In the present study, two questions were addressed empirically:
Within the aggregate New Mind client sample, what is the obtained rate of normative/reorganizational/total change over a course of neurofeedback therapy (standard and extended courses)? More specifically, when neurofeedback courses of varying lengths are examined, what are the expected percentages of change (a) toward the population norm(s and (b) percentage movement away from the population norms, after 20, 20-40, and 40-60 neurofeedback sessions?
What impact can clients’ general physiological health status be expected to have upon average rates of normative and reorganizational change?
Method:
Data Selection:
Archival data was draw from the New Mind Database System with key features in mind. All data was collected using the same type of amplifier. Data had been auto-artifacted using the New Mind auto-artifact system to assure data quality. This artifacting system does not involve algorithms that would distort phase or coherence (Thatcher, 2010). Clients had been subjected to standardized two channel bilateral protocols generated from the New Mind protocol generation algorithm (Soutar 2017). The protocol system is based on statistical analysis of all five major neuro-metric dimensions of magnitude, dominant frequency, phase, coherence and symmetry to determine the most statistically-deviant locations for training and an expert frequency analysis system that determined frequencies to be enhanced or suppressed (via amplitude training). This analysis system was based on protocols published in the neurofeedback research literature and based upon decades of clinical experience within the field. All protocols were magnitude-based protocols either enhancing or inhibiting deviant component bands based on the qEEG and utilizing what is presently recognized as methods of standard NFB (Coben et al, 2019).
qEEG map comparisons:
Client subjects whose data sets met eligibility for the present study had at least two qEEG data sets: pre-map and post-map. Some clients had undergone consecutive qEEGs, such as at 20 sessions, and/or 30 sessions, and/or 40 sessions, and/or at 60 sessions. Some clients may have had their post-map acquired at 20 sessions, whereas others may have had a postmap acquired at 30, 40, or 60 sessions.
The length of neurofeedback training varies, based upon the rate of progress (as assessed via trend screens and client weekly symptom tracking data), with this rate being potentially impacted by physiological, social/environmental, psychological, and lifestyle factors. Maps were analyzed in pair combinations. Quite a number of premap/postmap pairings were possible, such as
Pre-map vis post-map at 20 sessions.
Pre-map vis post-map at 30 sessions.
Pre-map vis post-map at 40 sessions.
Pre-map vis post-map at 60 sessions.
New Mind clinicians are trained, however, to acquire routine qEEGs every 15 to 20 sessions in order to ascertain progress, and to make protocol adjustments or substitutions. Hence, serial remaps (beyond the pre-map vs. post-map dyad) were typically conducted.
A standard course of neurofeedback lasts anywhere from 20 to 40 sessions. This leaves a number of map-remap possibilities:
Premap vs Remap1 (at 20 sessions)
Remap1 (at 20 sessions) vs. Remap2 (or post-map)at 30 sessions)
If neurotherapy is to be continued:
Remap2 (at 30 sessions) vs. Remap3/post-map at 40 sessions)
If an extended course of neurotherapy is necessary then:
Remap3 at 40 sessions) vs. Remap/Post-map at 60 sessions.
Client subjects were also divided into different health level categories based on the physiology checklist which statistically rates them against clinical norms. (See pdf in New Mind Maps ‘Help’ section). This was done to determine the effect of health on NFB training and its impact on map profiles.
Out of thousands of maps drawn from the original database sample we were able to find 477 cases that met all of these criteria. These cases included self-identified male and female genders spanning adults, adolescents and children between the ages of seven and ninety (ages 7 – 90 years) with a wide range of variety severity of cognitive/emotion/health problems.
Measures:
New Mind qEEG Interpretive System: The New Mind Assessment System is a qEEG based bio-psycho-social assessment instrument designed exclusively for the purposes of NFB and not for medical intervention, diagnosis, and not for psychological diagnosis, as well. It does not employ the DSM paradigm. It measures behaviors, symptoms, symptom levels, and relates them to probable brain locations and related functions. It then assesses electrophysiological status to confirm or disconfirm client symptom reports. It statistically evaluates NFB training locations and determines optimal locations for intervention as well as the best frequency training strategies and operantly-based reinforcement rates/ratios.
Clinicians trained in the New Mind system upload qEEG and questionnaire data for analysis and interpretation. These data includes 19 point qEEG brainmap raw data files for eyes-closed and eyes-open conditions, along with the Cognitive Emotional Checklist (CEC), the Interpersonal Style Inventory ((SI) , and the Physiological Symptom Checklist. The qEEG assessments were acquired using EEG amplifiers and a 19 channel qEEG electro-cap. The clients’ results are compared to a normative database using statistical analysis resulting in Z-score ratings of deviance or normalcy (above or below a population mean of Z = 0). The two sets of findings (reflecting eyes closed and eyes open conditions), summated client questionnaire responses, and statistically-derived qEEG measures are presented in the form of a dashboard that is quick and easy to read as well as a CSV file of measurements and qEEG head maps. This process is described in detail in ‘Holistic Neurointegration’ (Soutar, 2018).
The New Mind interpretive system (mapping, analysis, and protocol generation) relies purely on statistical analysis of five major neuro-metric dimensions to determine the most dysregulated areas of the brain as a system. This approach is based on neurophysics and is very empirical and unbiased. Complex multivariate statistical analysis is used to determine the best protocol locations and frequencies to be trained, exclusively for NFB clinicians and purposes, it is primarily a clinical (as opposed to research-designed) tool. Since protocols are based on carefully calculated neuro-metric analysis, they can be safely utilized quite flexibly. In NFB, we are looking for optimal, not “correct,” protocols. Because of the brain’s complexity, there can be no single solution—only many possible ones that occupy a complex solution space of high order.
qEEG Map Comparison Tool:
A map compare tool is also provided for ongoing assessment purposes. Any two sets of maps (collected at different time intervals during intervention) may be compared. Typically, the pre-map/post-map intervention map sets are compared. However, other data sets can be compared, such as Remap 1 (e.g. at 20 sessions) vs. Remap 2 (e.g. at 30 sessions) to ascertain rates of progress. This tool is based upon a detailed multivariate analysis, and the statistical algorithm is proprietary. Hence, clients can choose which maps they have done over time to compare and get a report providing side by side comparisons of each dimension of qEEG. Areas that move toward the norm, representing normalization of the distribution, are circled in green (termed ‘%normalization’’). Those that moved away-from-the-norm, representing deviance and re-organization processes, are circled in blue (termed ‘% re-organization’). The total movement toward the norm and away-from-the-norm is represented in at-a-glance meters labeled normalization and re-organization. A total normed percent change meter is also provided so that clinicians can get a sense of overall change in the EEG distribution because of training protocols, the number of training sessions between qEEG assessments, and ancillary complementary/alternative interventions (termed ‘% total change’ or ‘% global change’).
This ‘Compare Tool’ method is the first standardized system of its kind for NFB and for clinical work in general as far as we know. It allows clinicians to upload their autonomous client data to the system, and utilize the aggregate client database contributed to by hundreds of clinics to analyze and enhance the reliability and ecological validity of the system itself toward the goal of more effective outcomes.
The New Mind qEEG analysis system also provides auto-artifacting, which generates a consistent quality of artifact free data for analysis regardless of the practitioner’s experience and training in qEEG artifacting. This feature further enhances data quality and reliability for comparison purposes.
Physiological Symptom Checklist: The Physiological Symptom Checklist is comprised a list of physiological symptoms sampling domains of metabolic functioning. Each item is rated on a 5 point Likert scale in terms of increasing frequency/severity. Scoring of the checklist generates domain scores, and a total score. It allows the neuro-therapist to ascertain which metabolic systems, such as adrenal or thyroid, are correlated with abnormal qEEG. . It also provides advanced warning of which metabolic deficits might mitigate neurotherapeutic efforts to alter the qEEG distribution through NFB. Many such patterns, such as slowed elevated diffuse alpha (which tend to be associated with certain types of physiological problems) will not respond well to NFB until the client receives nutritional support (e.g. proper supplementation, dietary or medication changes, and other metabolic supports). The mean sample average on the Physiology questionnaire is 78, with a standard deviation of 75 and median of 53. Though strict cutoff scores are not used, a total score on the Physiological Checklist of 227 and higher suggests a potential problem which could confound training and likely to indicate a problem requiring referral to a health care provider for management. Though not prescriptive, the overall New Mind biopsychosocial qEEG interpretive system also provides indications of which supplements might assist the most in the effort to support metabolic systems and can be a useful adjunctive resource to other healthcare professionals in their decision making and diagnostic processes.
Results:
Data Analysis: qEEG brainmapping data sets where compared at various time intervals. The length of time to neurofeedback completion/termination was variable across client subjects, with some individuals (having reached their target goals for improvement) having completed intervention within 20 sessions, while other client subjects might not show significant adaptive change until 30, 40 to 60 sessions. Analyzing data via comparison of map sets (typically acquired at these time intervals) allowed for a more systematic analysis of the average rates of client progress over time/sessions.
Pre-Map = Pre-intervention qEEG (baseline)
Remap1 (e.g. qEEG remapping at session 20 or session 30)
Remap 2 (e.g. the third qEEG, also referred as Remap2, for example, at session 40 weeks).
Post-Map = Post-Intervention at whatever session length it occurred. This could be 20 weeks, 30 weeks, 40 weeks, 50 weeks, or 60 weeks, and will be specified in the data analyses.
The NewMind database system compares the neurometric values for magnitude, dominant frequency, coherence, phase and asymmetry obtained at each 10-20 location of any given map to a normative reference standard and determines the degree of deviance of each of those neurometric values from the norms of the normative reference in standard deviations.
The deviance of each pre and post map is computed accordingly and then compared. The percent change toward or away-from-the-normative reference in terms of standard deviations for each value is calculated.
The total percent change of all locations toward and away-from-the-normative reference is averaged for each neurometric dimension.
A grand average is then computed for all dimensions to provide a score of total change and consequently a score for total change toward the normative reference and a score for total change away from the reference.
The statistical output is generated below showing the mean rate of change for an average of twenty sessions. As previously noted, differences between all pre and post/remap values for each location were calculated in terms of percent of change in standard deviation. In this manner, we were able to provide a total percent of change in the pre and post maps. The Compare Tool also calculates percent of change in standard deviation; using this metric, we were able to partition the total (overall) % change into the percentages involving change toward the mean (% normalization) and the percentage of change away from the mean (% re-organization).

Based upon the statistics shown above, we were further able to calculate that the minimum amount of overall change was 14% and the maximum was 67%. These might at first seem to be unusual extremes, but they describe the range of change a neuro-therapist may expect to encounter in a typical pre-post/remap qEEG comparison. The average rate of client change was 38%. Having worked with clinics across the country over the last decade, the first author has observed that most first remaps or pre-post maps are in the 30%-40% change range, with a standard deviation range from 29% to 47% . The median rate of change was 38% and the modal rate of change was 34%, so the distribution is fairly Gaussian, as reflected in skewness and kurtosis values.
The aforementioned computed range of values (for the entire sample) closely approximates the typical values we encountered anecdotally as clinicians whenever clients were found to have reported good progress between qEEG assessments. Values between 47% and 56% have been found anecdotally to reflect atypically high amounts of change and efficacy, although the type of change taking place is also a critical consideration. Since the goal is to move the brain toward more normal ranges of neuro-metric values, the percentage of values moving toward the norm (% normalization) versus the percentage of values moving away-from-the-norm (% re-organization) are an important consideration. It is not uncommon to observe first remap values in the 40% range with respect to movement toward the norm (normalization) while on the other hand we usually see 60% of values moving away-from-the-norm (re-organization). It appears that these co-varying and complimentary change dimensions are necessary aspects of the neurotherapeutic change process and tend to compensate for each other as the brain moves through successive iterations of change toward the goal of increased stabilization and functionality. One might think of normalization as the ‘ying’ and re-organization as the ‘yang’ of change, which are optimally kept in functional balance.
In this sample, examination of the pre-post maps in the first 8 to 12 weeks (pre-qEEG to Remap1 qEEG), revealed an average total change rate of 40% , with an average normalization change rate of 34%, (range of 16% to 58%) and an average 44% re-organization rate (range of 18% to 61%). These normalization and re-organization rates turned out to be somewhat lower than expected. Yet the pattern suggests that it may be may be more common to see greater re-organization processes than normalization processes during the initial stages of neurofeedback (after the first qEEG remapping, or Remap1).
Early in the history of clinical applications of qEEG, some expert practitioners hypothesized that excess movement away-from-the-norm on the first post map may result from overtraining. However, Pascual-Leone’s (2005) research into compensation (as a normal process whereby neural re-organization must occur prior to normalization becoming more prominent) suggests an alternative hypothesis that may be better supported by the current findings. Since movement away-from-the-norm occurred at the same time that symptoms improved, it is more likely a re-organization process. In addition, the gradual increase in normalization (movement toward-the-norm) and a gradual decrease in re-organization (movement away-from-the-norm) suggests this is a natural ongoing process related to less disorder and greater stability. The fact that it is almost always the observed pattern (based upon years of experience of clinical observation and consensus by numerous clinicians) argues for the compensation theory as well.

Second Remap Changes
The 1st remap (Remap1) and 2nd remap (Remap2) qEEG maps were compared to designate change rate occurring during the interval separating the 20th and 30th sessions (which was about 14 to 20 weeks into the neurofeedback training). Again, the Total % change was found to be 40%, with % normalization rate at 32%, and re-organization rate at 46%. So again, normalization rate was lower than re-organization rate, but the difference between the normalization and re-organization was greater than in the previous (pre-map and Remap1) comparison (14% currently; 10% previously). Normalization decreased slightly (from 34% to 32%), possibly because the brain may have reached its immediate limits of change potential toward the norm. Re-organization continued to increase across sessions (44% to 46%).
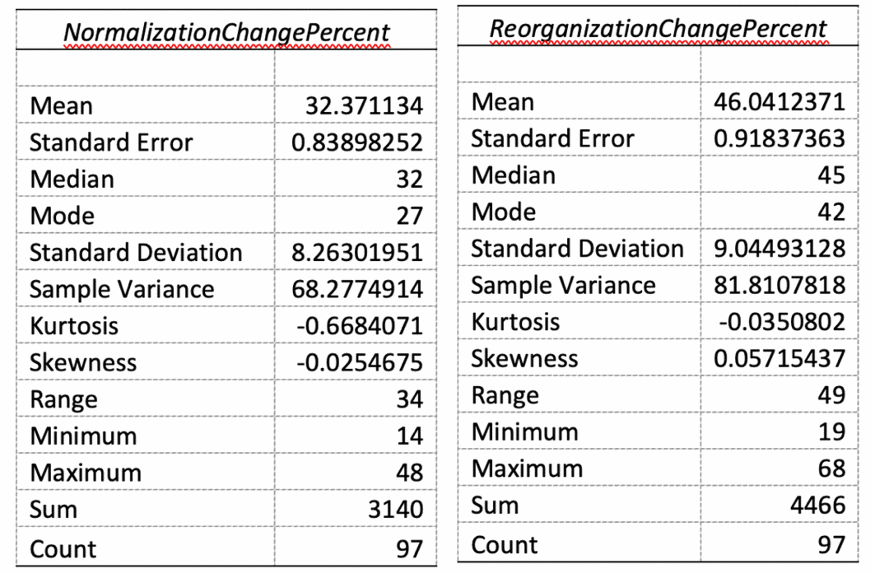
Third Remap Changes
In the interval spanning the 40th (Remap2) to 60th post/Remap3) session, the Total (overall) average percent change was 40%, whereas normalization change rate dropped down to 33%, and re-organization rate remained constant around 46%. Thus, normalization continues to be slightly lower in the second and third re-mappings, while re-organization remains about the same. The rate of normalization is slowing, perhaps plateauing. This suggests that the highest rate of % normalization change manifests earlier during training (while the qEEG is becoming better stabilized), then the normalization rate starts to slow or plateau. This trajectory is very similar to that which occurs in psychotherapy, where stabilization occurs first before additional slower incremental (but more lasting) changes occur. The aforementioned patterns are limited to maps with fairly average rates of change. By contrast, maps involving sub-average, or very high rates of change, may display different dynamics (a different change pattern and process).
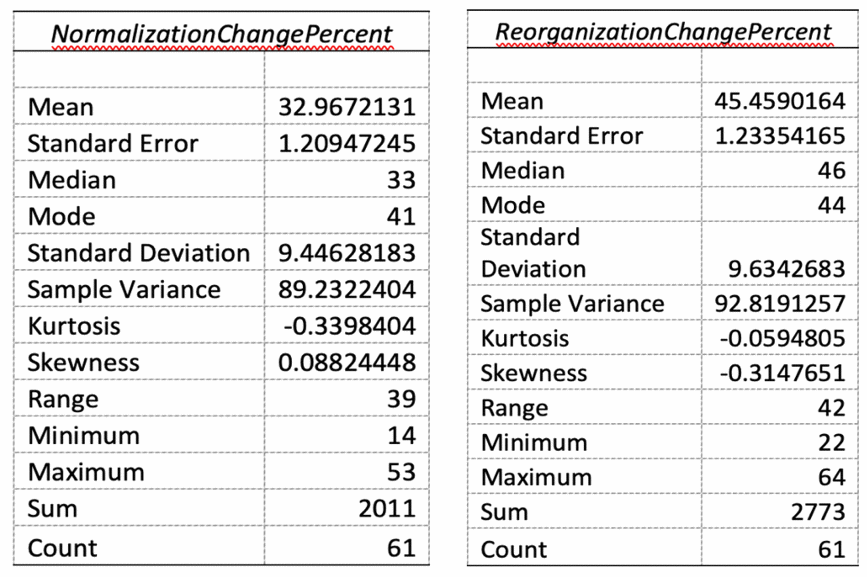
Further Analysis
In evaluating the data of an individual with a sleep disorder who was not engaged in NFB, we observed that morning to afternoon neuro-metric differences can vary significantly. Percent change can be as low as 15% when qEEGs are acquired in the morning and as high as 35% when qEEGs are acquired in the afternoon (e.g. when clients tend to get drowsy during mapping as the circadian rhythm reaches its low point during waking hours.) Correspondingly, Delta, Theta, and low frequency Alpha amplitudes increase, whereas mid to upper frequency Alpha amplitudes diminish as arousal decreases and drowsiness sets in. For this reason we recommend that all clinicians do pre and post maps in the morning. Some clinicians may have failed to follow protocol, which could be a minor confound in the data.
It has been observed clinically by a majority of practitioners (using the New Mind system) that, more frequently than not, normalization scores decrease as clients improve, and this appears to be supported by the data. The first author hypothesized originally that this may be due to the brain reaching an asymptotic level of change under the existing circumstances and with existing resources. The author and others were able to isolate 34 cases where individuals were trained for 60 sessions and had undergone pre-maps and post-map/remaps around both session 30 and session 60 , and these data were eligible for comparison. With a very high probability (p = .0024 and less than .05), we can conclude the data from this latter population is statistically significantly different from the more average population data previously presented.
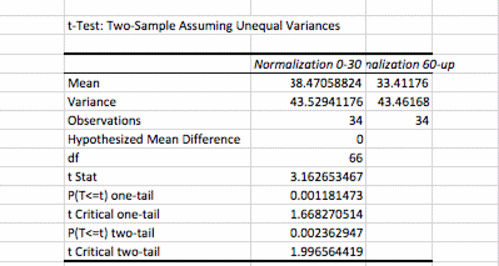
Although this may be the rule, there are striking exceptions and as in the case below it was observed that Overall Change diminished over the series of remaps suggesting at least a temporary limit to change. At the same time re-organization (here labeled as Retrograde Compensation) diminished eventually, while Normalization increased (here labeled as Anterograde Compensation).
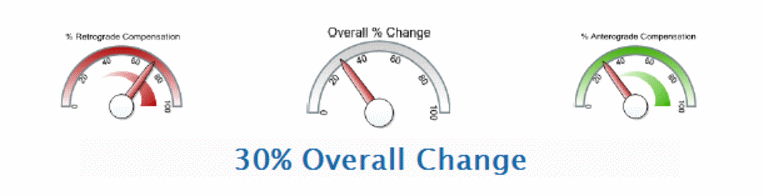
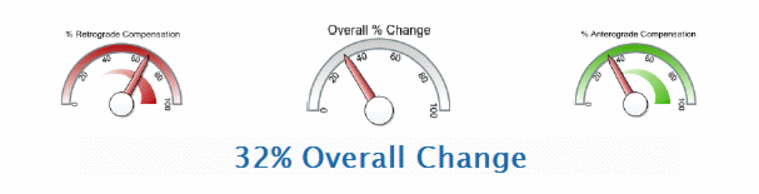
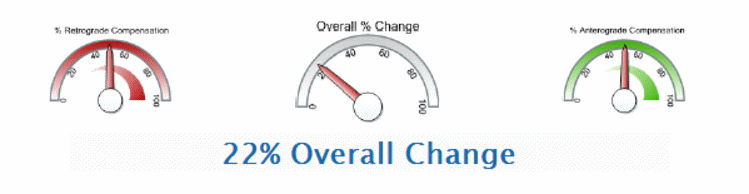
In other words, overall change diminished as values tend to move more toward greater percent of normalization, while moving away from higher re-organization values toward lower re-organization values. This would theoretically be an optimal outcome demonstrating positive progressive change toward a more normalized EEG distribution. This finding is consistent with research on neuroplasticity and compensation theory (Pascual-Leone, 2005). In both circumstances, clients improve. Why this is not more common remains a mystery, but it may represent the more rare super-optimal case. In either case, it would appear that the brain fatigues with respect to an excessively rapid rate of change due to normalization over time (perhaps by drawing upon its resources too heavily – at a faster rate than can be replenished – and thereby diminishing them).
Unexpectedly, rates of Change showed no appreciable difference between groups with average Physiological Functioning scores, versus Physiological Functioning scores over one SD above average, as well as no statistically significant difference between low Physiological Functioning scores and Physiological Functioning scores falling more than two SD units above the mean. Typically, clinicians find that weekly symptom tracking data frequently doesn’t reflect the expected changes in symptoms (symptoms do not significantly improve in the manner hoped for), as sessions progress among clients with very elevated Physiological Functioning scores and very high reported rates of health/medical problems. The preliminary conclusion is that while such clients’ brains may be more responsive to change, and may improve fairly rapidly via neurometric measures, positive change in their perceived physical health status and self- reported cognitive-emotional symptoms lags behind and takes longer to improve. Perhaps, this pattern reflects a progressive distraction with physical health-related symptoms and less satisfaction with NFB outcomes.
Discussion: This study was exploratory and preliminary in nature. It was designed to address two fundamental questions pertaining to effective neurofeedback training.
First, we wanted to obtain estimates, from our sample, of change across differing numbers of sessions, based upon point to point qEEG comparisons (pre-intervention qEEG data, as compared to serial Remap qEEGs, and post-intervention qEEG data (Post-map). Among those individuals with statistically average (plus or minus 1 standard deviation) neuro-metric index scores on the pre-intervention baseline qEEG, a pattern emerged over time (by way of comparison with a subsequent qEEG(s), whereby percent normalization (score movement toward the sample norms) gradually increased with an increased number of sessions, whereas re-organization processes started off significantly high, then gradually decreased or plateaued over sessions. However, exceptions were noted in subsets of individuals, where rates of normalization proceeded at very high rates, or at very low rates, and such individuals may show different dynamics or characteristics in their response to neurofeedback training than statistically more average individuals.
Self-related physiological problems did not appear to correlate significantly with qEEG-based rates of change, at least among persons whose scores fell plus or minus one standard deviation of the population norm. By contrast, among client subjects with very elevated Physiological problems scores, the qEEG metrics improved well before perceived health problems began to improve, and these individuals also showed a much slower rate of progress on weekly self-rated cognitive-emotional symptom tracking measures. Time of day when the qEEG was acquired was also relevant, and shifts in the qEEG data were apparent during the circadian cycle (e.g. slower arousal rate in the afternoon.) For this reason, data now support the common practice of acquiring qEEG’s during the last 3 hours of the morning in geographic areas with a day/night shift.
This appears to be the first study known to evaluate these questions, and to operationalize the chance process occurring during neurofeedback by using a Map Comparison analysis/tool to allow clinicians to assess % Total Change, % change due to movement toward the sample norm(s) also termed normalization, and % change away from the sample norm, also termed re-organization. Compensatory processes involving shifts away from the mean are a necessary and covarying component of change. After an initial bump upward in % normalization (as assessed at 20 weeks), % normalization rate then appears to proceed at a slow but steady rate among individuals whose qEEG neurometric values place them statistically within the average range (plus or minus 1 standard deviation of the population norm). Those individuals with more complex problems and/or other potential confounding influences, appear to have much slower rates of % normalization, with a much greater % reorganization as compared to % normalization.
Except among clients with very high rates of perceived physiological dysfunction, physiological symptoms were not found to be a detriment or impediment to adaptive change associated with neurofeedback training. Neuro-therapists can use the present preliminary data as a rough guideline to assist in predicting client response to sessions of neuro-therapy of varying length.
This study has the benefit of being naturalistic, and using what is considered the state- of-the-art assessment and reassessment measure, the qEEG, which yields neurometric data. Included as part of each qEEG brainmap are client self-report measures of cognitive and emotional functioning, interpersonal style, and physiological functioning. As part of the New Mind approach, neurotherapy clients are required to complete weekly trackers of the severity of their most distressing or problematic symptoms. Hence, the study has the advantage of a strong within-subjects longitudinal design. Although subject characteristics, symptom complaints, use of medication(s), and protocol length and duration vary (among other factors), by sampling broadly across a wide variety of clients and conditions, change variability that can ostensibly be attributed to the active intervention (neurofeedback) can be analyzed. Findings and conclusions are limited to clients seeking and completing either standard or extended courses of neurotherapy. The findings may generalize to this specific subpopulation, but not to treatment-seeking or non-treatment seeking individuals in the general population. Neurotherapists now have preliminary answers to several important questions, and can use that information to inform prospective clients, and estimate length and cost of treatment needed, based upon initial interview, client history, and pre-map neurometric derived from the qEEG, as well as psychometric data.
Clearly there are study limitations. This was not a controlled study. It was archival, exploratory, and empirically-focused (rather than being focused upon testing a theory or specific hypothesis). We posed open-ended questions (rather than specific predictions) regarding outcomes. and then evaluated these questions based upon statistical analysis of several sources of data (e.g. biometric and psychometric) derived from a large and broad spectrum database. Our study lacked any type of pre-assignment to conditions (such as treatment, no-treatment, or sham treatment). There was a clear selection bias in that data was derived from clients seeking neurotherapy, with the resources to do so, and having completed neurotherapy. There are sources of bias; ie. choice of protocol and length of treatment were not standardized across neurotherapy practitioners in different areas of the country being one potential source of bias. Further client differences and sources of potential biases include variable symptom constellations, variability in client diagnosis, age, gender, medication status, dietary and sleep patterns, etc.. It would have been ideal to have objective symptom ratings for each client rated by their Primary Care Physician, to complement the client-ratings on the Physiological questionnaire, but this was not feasible. And the study requires replication to strengthen reliability and validity. Perhaps this could be accomplished by the neuroscientist/practitioner developers of other major qEEG database. Until there are sources of grant funding to allow a more controlled study, analysis of qEEG data acquired at systematized time intervals/number of sessions represent the state of the art in this field.
Despite these potential shortcomings, it was hoped that the inclusion of a diverse and broad sample (based upon various clinics, regions, client demographics, various reported client problems, etc) minimized any sources of systematic bias in the sample, making it very representative of the average neurofeedback-seeking and intervention completing client. Following the initial clinical interview and assessment process, Neurotherapists now have preliminary empirical guidelines with which to advise/inform clients to help them plan and undertake the most cost-efficient and potentially therapeutically-effective course of intervention and assessment of progress toward goals. The present study fills a gap in neuroscience and qEEG/neurofeedback literature regarding expected outcomes of qEEG assessment and neurofeedback intervention. It is hoped that the study results help to guide neurotherapists and their clients, and to encourage neuroscience researchers to make independent efforts to replicate these methods and findings in the future.
References:
Coben, R., Hammond, D.C. & Arns, M. 19 Channel Z-Score and LORETA Neurofeedback: Does the Evidence Support the Hype?. Appl Psychophysiol Biofeedback 44, 1–8 (2019). https://doi.org/10.1007/s10484-018-9420-6
Freeman, Walter J. (2005). A field-theoretic approach to understanding scale-free neocortical dynamics. Biological Cybernetics, 92,6: 350-359.
Freeman, W. J., Ahlfors, S. P., Menon, V. (2009). Combining fMRI with EEG and MEG in order to relate patterns of brain activity to cognition. International Journal of Psychophysiology
Hammond, D., Walker, J., Hoffman, D., Lubar, J., Trudeau, D., Gurnee, R., Horvat, J. Standards for the use of quantitative electroencephalography (QEEG) in Neurofeedback: A position paper of the international society for neuronal regulation. Journal of Neuro-therapy 2004; 8(1): 5-27.
Hayes, A. M.; Laurenceau, J., Feldman, G., Strauss, J. L., & Cardaciotto, L. (2007). Change is Not Always Linear: The Study of Nonlinear and Discontinuous Patterns of Change in Psychotherapy, Clinical Psychology Review, 27, 715-723 doi:10.1016/j.cpr.2007.01.008.
Pascual-Leone, A., Amedi, A., Fregni, F., and Merabet, L. B. (2005) The Plastic Human Brain Cortex. Annual Review of Neuroscience, 28, 377-401.
Soutar, R. (2017). Perspective and method for a qEEG based two-channel bi-hemispheric compensatory model of neurofeedback training. In T. Collura & J. Frederick (Eds.), Handbook of clinical QEEG and neuro-therapy (pp. 387-403). New York, NY: Routledge
Soutar, R (2014). An introductory perspective on the emerging application of qEEG in neurofeedback. In D. Cantor & J. Evans (Eds.), Clinical neuro-therapy : Application of techniques for treatment (pp. 19-50). New York, NY: Elsevier.
Soutar, R. (2018). Holistic Neurointegration: The New Mind Model. A Bio-psycho-social qEEG Guided Method. (Unpublished Manual).
Thatcher, R (2010). Journal of Neuro-therapy , Validity and Reliability of Quantitative Electroencephalography 14: 122-152. DO – 10.1080/10874201003773500